Loading...
Aluminium (ALI) History
[[ data.name ]]
[[ data.ticker ]]
[[ data.price ]] [[ data.change ]] ([[ data.changePercent ]]%)
Low: [[ data.low ]]
High: [[ data.high ]]
Aluminium History
Although aluminium is a very rare element, its existence in pure metallic form evaded humanity for centuries due to the complexities of extracting it from ore. However, the use of aluminium compounds, like alum, has been documented as far back as the 5th century BCE, particularly in dyeing processes. Alum's importance in dyeing elevated it to a valuable trade commodity during the Middle Ages. It wasn't until the Renaissance that scholars began to suspect alum contained an unknown element. By the Age of Enlightenment, they determined this element, alumina, was an oxide of a new metal. In 1825, Danish physicist Hans Christian Ørsted, followed by German chemist Friedrich Wöhler, formally introduced the world to aluminium.
The initial challenge of refining aluminium made it more expensive than gold and therefore impractical for widespread use. This high cost barrier began to crumble in 1856 with the innovation of the first industrial production process by French chemist Henri Étienne Sainte-Claire Deville. Accessibility increased dramatically with the independent development of the Hall–Héroult process in 1886 by French engineer Paul Héroult and American engineer Charles Martin Hall, followed closely by the 1889 Bayer process, pioneered by Austrian chemist Carl Joseph Bayer. These groundbreaking methods revolutionized aluminium production and remain the industry standard today.
The ability to mass-produce aluminium unleashed its potential, leading to its widespread adoption across industries and everyday life. Its lightweight and corrosion-resistant properties proved invaluable in engineering and construction, securing its role as a critical resource in aircraft production during World Wars I and II. Consequently, global aluminium production experienced explosive growth, skyrocketing from a mere 6,800 metric tons in 1900 to a staggering 2,810,000 metric tons in 1954. This surge propelled aluminium to surpass copper as the world's leading non-ferrous metal.
The latter half of the 20th century witnessed aluminium's expanded use in the transportation and packaging sectors. However, this progress came at a cost, as environmental concerns surrounding aluminium production began to surface. Consequently, aluminium recycling gained traction as a more sustainable practice. The 1970s marked aluminium's entry into the commodity market, coinciding with a production shift from developed to developing nations. By 2010, China had become a dominant player in both the production and consumption of aluminium. Global production continued its upward trajectory, reaching 58,500,000 metric tons in 2015, cementing aluminium's position as the undisputed leader in non-ferrous metal production.
Early History
Alum, a compound of aluminium, has a long and storied history. Ancient civilizations, as early as the 5th century BCE, recognized its value. The Greek historian Herodotus documented its use as a mordant in dyeing, a medicinal substance, a chemical milling agent, and a fire retardant coating for wood, particularly in fortifying structures against arson. While the use of alum was well-known, aluminium metal itself remained undiscovered.
Intriguingly, the Roman writer Petronius, in his work Satyricon, tells of a unique glass presented to the emperor. Remarkably resilient, the glass would deform under impact rather than shatter and could be reshaped with a hammer. Fearful of devaluing gold, the emperor, upon learning of the inventor's exclusive knowledge, had him executed to suppress the discovery. Variations of this account appear in works by Pliny the Elder and Cassius Dio, though its authenticity is debated. Some speculate that this resilient glass may have been an early form of aluminium. Further evidence suggests that aluminium alloys might have been produced in China during the Jin Dynasty (266-420 CE).
Following the Crusades, alum became a significant commodity in international trade, particularly essential to the European textile industry. While small alum mines operated in Catholic Europe, the Middle East remained the primary source, with trade primarily occurring across the Mediterranean Sea. This changed in the mid-15th century when the Ottoman Empire significantly increased export taxes on alum. Shortly after, abundant alum deposits were discovered in Italy. Capitalizing on this discovery, Pope Pius II banned all alum imports from the East, leveraging the profits from this new source to finance a war against the Ottomans. Italian alum became a cornerstone of European pharmaceuticals, but the papal government's pricing policies eventually drove other nations to seek their own sources. Consequently, large-scale alum mining spread to other European regions during the 16th century.
The enigmatic nature of alum puzzled scholars at the dawn of the Renaissance. It wasn't until around 1530 that Swiss physician Paracelsus distinguished alum from vitriols (sulphates), proposing its classification as an earth salt. In 1595, German physician and chemist Andreas Libavius, through his experiments, demonstrated that alum, green vitriol, and blue vitriol shared a common acid but differed in their earthly constituents. He christened the unknown earth found in alum "alumina". In 1702, German chemist Georg Ernst Stahl postulated that alum's base shared similarities with lime or chalk, a misconception that persisted in scientific circles for the next half-century. Friedrich Hoffmann, a German chemist, challenged this view in 1722, suggesting that alum's base was a distinct earth altogether. This notion was furthered by French chemist Étienne Geoffroy Saint-Hilaire in 1728, who, while mistakenly believing that burning the earth yielded silica, asserted that alum arose from the interaction of an unknown earth with sulphuric acid. It took until 1785 for German chemist and pharmacist Johann Christian Wiegleb to rectify Geoffroy's error, demonstrating that, contrary to prevailing beliefs, the earth of alum could not be synthesised from silica and alkalis. Adding to this growing body of knowledge, French chemist Jean Gello, in 1739, proved the identical nature of the earth present in clay and the earth produced by the reaction of an alkali with alum. Further cementing the distinctiveness of alum's base, German chemist Johann Heinrich Pott, in 1746, demonstrated that the precipitate resulting from the addition of an alkali to an alum solution differed from both lime and chalk.
A breakthrough came in 1754 when German chemist Andreas Sigismund Marggraf successfully synthesised the earth of alum. His method involved boiling clay in sulphuric acid and introducing potash. He observed that the addition of soda, potash, or any alkali to a solution of this newly synthesised earth in sulphuric acid resulted in the formation of alum. Marggraf, observing its solubility in acids after drying, characterized this earth as alkaline. His work also extended to describing the salts of this earth, including chloride, nitrate, and acetate. In 1758, French chemist Pierre Macquer drew a comparison between alumina and metallic earths, a view echoed by his fellow countryman, chemist Théodore Baron d'Hénouville, in 1760, who expressed confidence in alumina's identity as a metallic earth.
Swedish chemist Torbern Bergman, in 1767, furthered the understanding of alum by synthesising it through two distinct methods: boiling alunite in sulphuric acid and adding potash to the solution, and reacting potassium sulphates with the earth of alum. Through these experiments, he established alum's identity as a double salt. Adding to the growing clarity, Swedish German pharmaceutical chemist Carl Wilhelm Scheele, in 1776, demonstrated that both alum and silica shared their origin in clay and that alum was devoid of silicon. By 1782, the renowned French chemist Antoine Lavoisier classified alumina as an oxide of a metal, proposing that its affinity for oxygen was so potent that no known reducing agents could break the bond.
In 1815, Swedish chemist Jöns Jacob Berzelius put forward the formula AlO3 for alumina. However, it was German chemist Eilhard Mitscherlich who, in 1821, established the correct formula as Al2O3. This correction proved instrumental in Berzelius's subsequent determination of the metal's accurate atomic weight: 27.
Industrial Production
In 1854, at the Paris Academy of Sciences, French chemist Henri Étienne Sainte-Claire Deville unveiled a groundbreaking industrial method for producing aluminium. His process involved the reduction of aluminium chloride using sodium, a more practical and cost-effective alternative to the potassium employed by Wöhler. This innovation enabled Deville to successfully create an ingot of the metal. Intrigued by its potential military applications, Napoleon III pledged substantial financial support to Deville's research, hoping to equip the French army with lightweight and resilient weapons, helmets, armour, and other equipment fashioned from this new and lustrous metal. Although not yet ready for public display, aluminium's allure was such that Napoleon is said to have hosted a banquet where esteemed guests dined with aluminium utensils, a privilege denied to others who had to settle for gold.
The Exposition Universelle of 1855 marked the first public exhibition of twelve small aluminium ingots. Dubbed "the silver from clay" due to its striking resemblance to silver, the metal garnered significant interest and sparked widespread speculation about its potential applications in art, music, medicine, culinary arts, and tableware. Avant-garde writers of the era, including Charles Dickens, Nikolay Chernyshevsky, and Jules Verne, envisioned a future shaped by aluminium. However, the reception was not without its critics. Some newspapers dismissed the initial hype, claiming that the exhibited quantity, a mere kilogram, fell short of expectations and cast doubt on the revolutionary impact of the metal. Despite this scepticism, the exposition ultimately paved the way for aluminium's commercialisation. That year, it entered the market priced at 300 francs per kilogram. By the subsequent Paris fair in 1867, aluminium wire, foil, and a new alloy—aluminium bronze—were on display, showcasing the metal's versatility and its economical production cost, impressive corrosion resistance, and desirable mechanical properties.
Early attempts to produce aluminium commercially were hampered by several factors. Manufacturers were hesitant to divert resources away from established metals like iron and bronze, preferring to focus on these known and readily marketable materials. Furthermore, the aluminium produced at this time was often impure, its properties varying significantly between batches. This inconsistency created reluctance within industries to embrace the new metal.
Despite these challenges, Deville and his partners established the world's first industrial aluminium production facility in Rouen in 1856. This smelter was subsequently relocated several times, ultimately settling in Salindres. By 1858, Deville had refined his process, utilising bauxite as the primary source of alumina. He later sold his aluminium interests to Henri Merle's Compagnie d'Alais et de la Camargue, a company that would go on to dominate the French aluminium market for decades.
While Deville's process represented a significant advancement, it was not without its limitations. Output remained relatively low, reaching just 1.8 metric tons by 1872. Demand for aluminium was also limited, with the metal often being compared to silver and primarily used for decorative items and jewellery.
Throughout the 1880s, new production sites emerged, each attempting to refine the process and improve the purity of the aluminium produced. British engineer James Fern Webster achieved notable success in 1882, his method producing significantly purer aluminium than Deville's. In America, William Frishmuth streamlined production, combining the production of sodium, alumina, and aluminium into a single process, while Hamilton Castner's innovations in sodium production significantly reduced the cost of aluminium. Despite these strides, the widespread adoption of aluminium remained elusive, hindered by high production costs and limited industrial applications.
Aluminium’s Mass Usage
The declining price of aluminium in the late 19th century led to its widespread adoption in a variety of everyday objects, from jewellery and eyeglass frames to optical instruments. The late 19th and early 20th centuries witnessed a surge in the use of aluminium. Cookware made from this lightweight metal began to replace traditional copper and cast iron pots and pans in the early 1900s, coinciding with the rise in popularity of aluminium foil. Metallurgists discovered that alloying aluminium with other metals enhanced its strength without compromising its low weight. This led to the development of alloys like aluminium bronze, used extensively in shipbuilding and aviation for its flexibility and strength. The invention of duralumin in 1903 further propelled aluminium's use in aviation, notably in the construction of the Wright Flyer's engine.
The dawn of the 20th century saw the emergence of aluminium recycling, a practice that quickly gained traction. Aluminium's ability to be recycled repeatedly without degradation made it an ideal candidate for this process. Initially, only aluminium that had not reached consumers was recycled. However, the outbreak of World War I dramatically escalated the demand for aluminium, particularly for lightweight yet robust aircraft components. Governments across the globe invested heavily in aluminium production, subsidising factories and bolstering electrical grids to meet the soaring demand. Global output surged from a modest 6,800 metric tons in 1900 to over 100,000 metric tons by 1916. This surge, however, couldn't keep pace with wartime requirements, leading to a significant increase in aluminium recycling.
The post-war years witnessed a dip in aluminium production, followed by a period of rapid growth. The real price of aluminium steadily decreased throughout the first half of the 20th century, plummeting from $14,000 per metric ton in 1900 to $2,340 in 1948, with the exception of a sharp spike during World War I. This affordability, coupled with its abundance, led to its adoption in various applications. Germany, grappling with hyperinflation in 1919, began substituting its silver coins with aluminium counterparts. By the mid-20th century, aluminium had become ubiquitous, firmly established as a staple in households worldwide.
The 1930s marked a turning point for aluminium as it entered the realm of civil engineering, employed in both structural and interior applications. Simultaneously, its use in military engineering, particularly in aircraft and tank engines, expanded. The transportation industry benefited from aluminium's lightweight properties with the introduction of aluminium freight cars in 1931, enabling greater cargo capacity.
Despite the growth of recycling, primary aluminium remained superior due to challenges in maintaining consistent chemistry and effectively removing impurities during the recycling process. Factors like fluctuating energy prices also impacted recycling rates. For example, when energy prices in the United States fell in the late 1930s, producing primary aluminium using the energy-intensive Hall–Héroult process became more economically viable, leading to a decline in aluminium recycling. Nevertheless, by 1940, the mass recycling of post-consumer aluminium had become a reality.
The Second World War saw a surge in aluminium production, surpassing one million metric tons for the first time in 1941. Its use in aircraft manufacturing made it a crucial strategic asset. The significance of aluminium was such that when Alcoa, the dominant force in American aluminium production at the time, hesitated to increase output, the US Secretary of the Interior famously declared in 1941, "If America loses the war, it can thank the Aluminum Corporation of America". Germany, the leading aluminium producer in 1939, viewed this advantage as pivotal to their war effort. Initially symbolic of decline, aluminium coins had, by 1939, become a representation of power. However, 1941 saw their withdrawal from circulation to conserve the metal for military purposes. Following its entry into the war in 1940, the United Kingdom initiated a large-scale aluminium recycling programme, with the Minister of Aircraft Production urging the public to contribute any available household aluminium for aircraft construction. The Soviet Union, between 1941 and 1945, received 328,100 metric tons of aluminium from its allies, crucial for their aircraft and tank engine production. It is estimated that without these supplies, Soviet aircraft output would have been halved.
Although global production declined for a brief period after the war, it soon resumed its rapid ascent. By 1954, world output reached 2,810,000 metric tons, exceeding that of copper and establishing aluminium as the most produced non-ferrous metal, second only to iron in overall metal production.
The Aluminium Age
The launch of Earth's inaugural artificial satellite in 1957, constructed from two conjoined aluminium hemispheres, marked the beginning of aluminium's extensive use in spacecraft. Interestingly, the aluminium can, first produced in 1956, found its initial application as a beverage container in 1958. The 1960s saw aluminium being utilised in the production of wires and cables. From the 1970s onwards, its high strength-to-weight ratio made it a popular choice in the construction of high-speed trains and contributed to its increasing presence in the automotive industry.
By 1955, the global aluminium market was dominated by six major players: Alcoa, Alcan (which originated from Alcoa), Reynolds, Kaiser, Pechiney (a merger of Compagnie d'Alais et de la Camargue, who acquired Deville's smelter, and Société électrométallurgique française, who employed Héroult), and Alusuisse (successor to Héroult's Aluminium Industrie Aktien Gesellschaft). These companies collectively held an 86% market share. For almost three decades after 1945, aluminium consumption experienced a near-constant 10% annual growth, driven by its expanding use in building applications, electric cables, basic foils, and the aircraft industry. The advent of aluminium beverage cans in the early 1970s further propelled this growth. This surge in production, coupled with technological advancements and reduced extraction and processing costs, contributed to a decline in the real price of aluminium until the early 1970s. By 1973, the real price had fallen to $2,130 per metric ton (in 1998 United States dollars). Global aluminium production surpassed 10,000,000 metric tons for the first time in 1971.
During the late 1960s, governments began to recognise the environmental impact of industrial waste. Regulations were implemented to encourage recycling and waste disposal. Söderberg anodes, while cost-effective in terms of capital and labour for anode baking, were environmentally detrimental due to the challenges in capturing and disposing of baking fumes. Consequently, these fell out of favour, and the industry shifted back to pre-baked anodes. In an effort to pre-empt potential restrictions on aluminium cans, the aluminium industry began promoting their recycling. This spurred the recycling of post-consumer aluminium. In the United States, for instance, the recycling rate for this type of aluminium increased 3.5 times from 1970 to 1980, and a further 7.5 times by 1990. Rising primary aluminium production costs during the 1970s and 1980s also contributed to the growth of aluminium recycling. Additionally, advancements in composition control and refining technology narrowed the quality gap between primary and secondary aluminium.
The 1970s saw aluminium become a traded commodity due to increased demand. It was listed on the London Metal Exchange, the world's oldest industrial metal exchange, in 1978. From that point onwards, aluminium has been traded in US dollars, with its price fluctuating alongside currency exchange rates. Several factors, including the need to exploit lower-grade deposits, escalating energy and bauxite input costs, currency fluctuations, and greenhouse gas regulations, contributed to a rise in the net cost of aluminium. Consequently, the real price of aluminium increased throughout the 1970s.
The rising real price of aluminium, coupled with changes in tariffs and taxes, led to a shift in global production shares. In 1972, the United States, the Soviet Union, and Japan collectively accounted for nearly 60% of global primary production and a similar proportion of primary aluminium consumption. However, by 2012, their combined share had dwindled to slightly over 10%. This production shift, which began in the 1970s, saw production relocate from the United States, Japan, and Western Europe to regions such as Australia, Canada, the Middle East, Russia, and China. These regions offered lower production costs due to cheaper electricity prices and favourable government policies, including tax breaks and subsidies. Technological advancements, lower energy and alumina prices, and a strong US dollar contributed to a decline in production costs during the 1980s and 1990s.
The dawn of the 21st century saw the combined share of the BRIC nations (Brazil, Russia, India, and China) in primary production surge from 32.6% to 56.5%, and their share of primary consumption rise from 21.4% to 47.8%. China, in particular, amassed a significant portion of global production due to abundant resources, inexpensive energy, and government incentives. The country's share of consumption also skyrocketed from a mere 2% in 1972 to a staggering 40% in 2010. The only other country to hold a double-digit percentage was the United States at 11%, with no other country exceeding 5%. Transportation, engineering, construction, and packaging were the primary sectors for aluminium consumption in the United States, Western Europe, and Japan.
Escalating energy, alumina, and carbon (used in anodes) prices exerted upward pressure on production costs in the mid-2000s. This was exacerbated by shifts in currency exchange rates, particularly the weakening of the US dollar and the strengthening of the Chinese yuan. The latter became increasingly significant as a large proportion of Chinese aluminium was relatively inexpensive.
Despite these cost pressures, global aluminium output continued its upward trajectory, reaching a record 63,600,000 metric tons in 2018 before experiencing a slight dip in 2019. Aluminium production now surpasses that of all other non-ferrous metals combined. In 2019, the real price of aluminium (in 1998 US dollars) stood at $1,400 per metric ton, which equates to $2,190 per ton in today's currency.
Swap long | [[ data.swapLong ]] points |
---|---|
Swap short | [[ data.swapShort ]] points |
Spread min | [[ data.stats.minSpread ]] |
Spread avg | [[ data.stats.avgSpread ]] |
Min contract size | [[ data.minVolume ]] |
Min step size | [[ data.stepVolume ]] |
Commission and Swap | Commission and Swap |
Leverage | Leverage |
Trading Hours | Trading Hours |
* The spreads provided are a reflection of the time-weighted average. Though Skilling attempts to provide competitive spreads during all trading hours, clients should note that these may vary and are susceptible to underlying market conditions. The above is provided for indicative purposes only. Clients are advised to check important news announcements on our Economic Calendar, which may result in the widening of spreads, amongst other instances.
The above spreads are applicable under normal trading conditions. Skilling has the right to amend the above spreads according to market conditions as per the 'Terms and Conditions'.
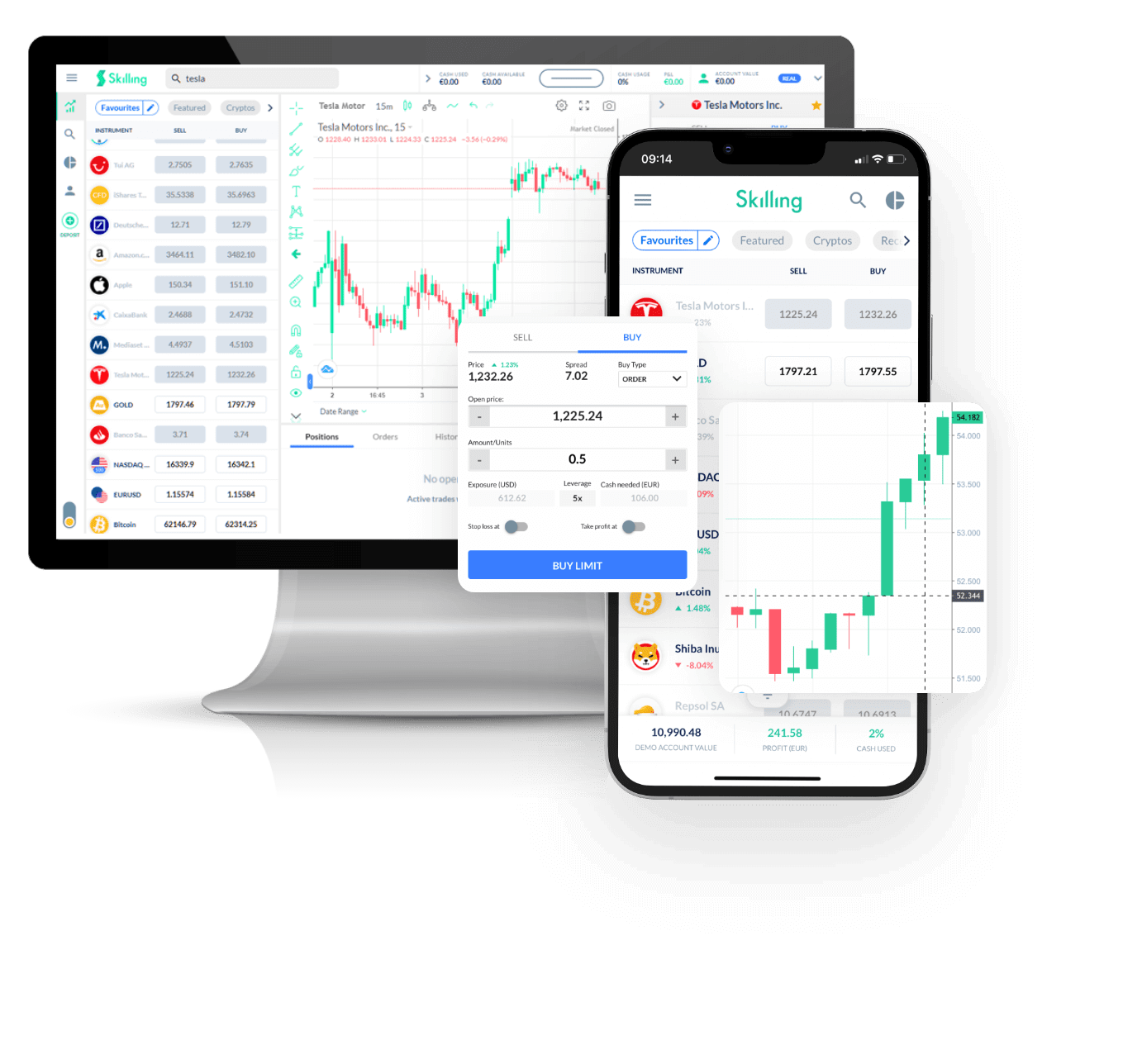
Trade [[data.name]] with Skilling
Take a view on the commodity sector! Diversify with a single position.
- Trade 24/5
- Tight spreads
- Average Execution at 5ms
- Easy to use platform
FAQs
How does trading aluminium CFDs work?
+ -
Trading aluminium CFDs involves speculating on the price movements of aluminium without owning the physical metal. A CFD (contract for difference) is a derivative instrument that allows traders to profit from the difference in the price of aluminium between the opening and closing of the trade.
Traders could go long (buy) if they anticipate the price will rise or go short (sell) if they believe it will fall. When trading aluminium CFDs, traders enter into a contract with a broker and make a profit or loss based on the difference between the entry and exit prices. It's important to note that CFD trading carries risks, including the potential for losses exceeding the initial investment.
What factors affect the price of Aluminium?
+ -
Several factors could impact the price of aluminium. Firstly, global supply and demand dynamics play a crucial role. If the demand for aluminium exceeds the available supply, prices tend to rise, and vice versa. Economic conditions, such as GDP growth, industrial production, and construction activity, also influence the prices. Additionally, geopolitical events like trade disputes or political instability could affect prices by disrupting supply chains or imposing tariffs.
Energy costs are also significant as aluminium production requires substantial energy inputs. Currency exchange rates also play a role since aluminium is priced in USD, fluctuations in currencies could impact its cost. Lastly, government policies and regulations regarding production, trade, or environmental standards could influence its prices.
How do I analyze the trend of aluminium prices?
+ -
To analyze the trend of aluminium prices, several factors should be considered. Firstly, historical price data may be examined using charts and graphs to identify patterns and trends over time. Technical analysis tools such as moving averages, support and resistance levels, and momentum indicators could also help identify potential price movements.
Additionally, staying informed about market news, industry reports, and forecasts from reputable sources could provide valuable insights into supply and demand dynamics and macroeconomic factors affecting its prices. It's important to consider both fundamental analysis, which examines factors like global economic conditions and industry trends, and technical analysis when analyzing the trend of the prices.
Why Trade [[data.name]]
Make the most of price fluctuations - no matter what direction the price swings and without the restrictions that come with owning the underlying asset.
CFD
Actual Commodities
Capitalise on rising prices (go long)
Capitalise on falling prices (go short)
Trade with leverage
Trade on volatility
No commissions
Just low spreads
Manage risk with in-platform tools
Ability to set take profit and stop loss levels